

DAM50
DAM50 Bio-Amplifier, Low noise, battery-operated amplifier
- Overview
- Specifications
- Accessories
- Citations
- Related Products
Overview
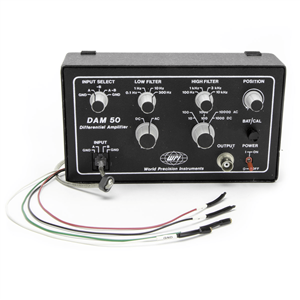
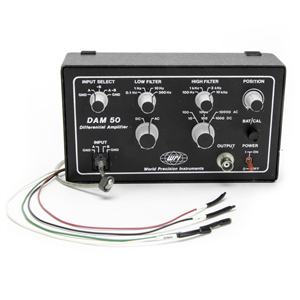
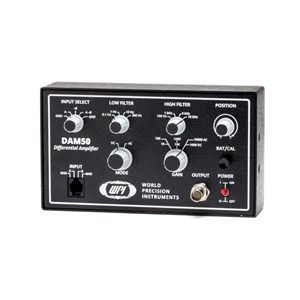
There are 3 images available to view - click to enlarge and scroll through the product gallery.
DAM Amplifier Series Datasheet
/ Download as PDF
DAM50 Instruction Manual
/ Download as PDF
Benefits
- Very low internal noise
- Ultra quiet DC power supply — no AC required
- Intrinsic low susceptibility to ground loops
- Small footprint
- Cost-effective
- Electrostatic Discharge?Protection!
Applications
- Amplifying biopotentials from metal electrodes
- Brain slice field stimulation
- EAG (Electroantennogram)
- ERG (Electroretinogram)
Features
WPI’s DAM series amplifier’s are well known as a standard of the industry for extracellular potential amplification. These battery powered bio-amplifiers are designed with a compact chassis profile that enables the user to locate the unit closer to the preparation and thereby minimize long lead lengths which contribute to noise. Each amplifier is equipped with selectable high and low filters, and a position control to offset galvanic potentials which may develop during recording. A choice of models offer additional features that are useful for certain applications.
Portability
DAM series amplifiers can be used as standalone units on any tabletop, or use optional clamp-mounting hardware to locate them conveniently within the work area. Alternatively, a pair of amplifiers can be mounted into a standard equipment rack with a rack mount kit (#3484). A variety of hook up accessories are available to configure your application.
Using a DAM50 for EEG Recordings in Rodents
A low-noise amplifier like the DAM50 is an excellent choice for EEG recording in rodents. WPI’s amplifiers were engineered for the bio-medical researcher. While 20-30μV of noise is common in bio-amplifiers, WPI’s DAM series amplifiers generate 0.4μV RMS (root mean squared) at 0.1-100Hz. (That’s equal to about 2μV peak to peak.) This setup shows one way such recordings could be made. The RC1 electrode works well for rats, and the EP1 is more suitable for mouse cranial application.
Rat Cranial Applications
The RC1 electrode comes with a 4' unterminated wire attached. That needs to be soldered to the green-white ground wire of the 5470 cable.
- The red wire from the 5470 cable connects with the non-inverting input on the amplifier, and the black wire connects with the inverting wire on the amplifier.
- Solder a Profile A tungsten electrodes to the red and black wires.
- For best results, seal the solder joints with a heat shrink tubing.
- Affix the Profile A electrodes in the M3301EH electrode holders as shown in the images below.
- Position the electrode holders into the two positions on the MD4R micromanipulator.
- Position the electrodes so that the tips of the electrodes are nearly touching. These electrodes can then be precisely positioned in a rodent's brain.
Mouse Cranial Applications
The EP1 is a smaller electrode and is better suited for small rodents than the RC1 electrode. This application uses the same setup as the first one shown. In addition to the EP1 electrode, you will also need a length of wire to connect the green-white ground wire from the WPI #5470 cable with the EP1 electrode.
Feature Comparision Chart
DAM50 | DAM80 | |
Input Mode | AC/DC | AC |
Input Configuration | Differential/Single Ended | Differential |
Gain Range | 100-10,000 (AC) 10-1,000 (DC) |
100 - 10,000 (AC) |
High/Low Filters | Yes | Yes |
Offset Position Control | Yes | Yes |
Current Generator | No | Yes |
Remote Active Headstage | No | Yes |
Output Connection | BNC | 3.5 mm mini phone |
Standard Input Connection | unterminated wire | Mini banana |
Power Supply | (2) 9V alkaline batteries | (2) 9V alkaline batteries |
Specifications
INPUT IMPEDANCE | 1012 Ω, common mode and differential |
INPUT LEAKAGE CURRENT | 50 pA (typical) |
MAX. DC DIFFERENTIAL SIGNAL | ±2.5 V (DAM 50) |
GAIN | AC: 100x, 1000x, 10000x, DC: 10x, 100x, 1000x (DAM50) |
COMMON MODE REJECTION RATIO | 100dB @ 50/60 Hz |
INPUT CAPACITANCE | 20 pF |
AC MODE NOISE | 0.4 μV RMS (2uV p-p) 0.1-100 Hz |
AC MODE NOISE | 2.6 μV RMS (10uV p-p) 1 Hz-10 kH |
DC MODE NOISE (DAM50) | (DAM50) 7.5uV RMS (30uV p-p) 3-10 kHz |
BANDWIDTH FILTER SETTINGS:AC Mode | Low frequency, 0.1, 1, 10, 300 Hz |
BANDWIDTH FILTER SETTINGS: DC Mode(DAM50) | High frequency, 0.1, 1, 3, 10 kHz |
OUTPUT CONNECTORS | BNC |
OUTPUT VOLTAGE SWING | ±8 V |
OUTPUT IMPEDANCE | 470 Ω |
BATTERY TEST | Audible tone |
CALIBRATOR SIGNAL | 10 Hz square wave |
POSITION | Approximately 250 mV |
EXTERNAL COMMAND | Input Voltage ±10 V commands |
AC or DC current waveform | ±50μA max. amplitude @ 200 KΩ |
BATTERIES | 2 x 9 V alkaline (included) |
DIMENSIONS:DAM50 | 20.3 x 10.2 x 4.4 cm |
SHIPPING WEIGHT | 1.6 kg |
The following bandwidth charts for the DAM50 show the response of the amplifier when various filters and gains are used.
GAINS: This chart shows the standard 3dB frequency cutoff at maximum filter band pass.
FILTERS: Various low pass and high pass filters were applied at the AC x1000 gain setting to show the bandwidth of and actual DAM50 amplifier.
Accessories
Citations
Kim, E. Y., & Virginia, W. (n.d.). Effect of Growth Hormone on Hippocampal Synaptic Function during Sleep Deprivation By.
full-text. (n.d.).
Dai, J., Brooks, D. I., & Sheinberg, D. L. (n.d.). Supplemental Information Optogenetic and Electrical Microstimulation Systematically Bias Visuospatial Choice in Primates.
Škorjanc, A., & Belušic, G. (n.d.). How We Teach: Classroom And Laboratory Research Projects Investigation of blood flow and the effect of vasoactive substances in cutaneous blood vessels of Xenopus laevis.
Liu, Y., Wang, Y., Zhu, G., Sun, J., Bi, X., & Baudry, M. (2016). A calpain-2 selective inhibitor enhances learning & memory by prolonging ERK activation. Neuropharmacology, 105, 471–477. http://doi.org/10.1016/j.neuropharm.2016.02.022
Ztaou, S., Maurice, N., Camon, J., Guiraudie-Capraz, G., Kerkerian-Le Goff, L., Beurrier, C., … Amalric, M. (2016). Involvement of Striatal Cholinergic Interneurons and M1 and M4 Muscarinic Receptors in Motor Symptoms of Parkinson’s Disease. Journal of Neuroscience, 36(35).
Kentish, S. S. J., Frisby, C. L., Kritas, S., Li, H., Hatzinikolas, G., O’Donnell, T. A., … Ahern, G. (2015). TRPV1 Channels and Gastric Vagal Afferent Signalling in Lean and High Fat Diet Induced Obese Mice. PloS One, 10(8), e0135892. http://doi.org/10.1371/journal.pone.0135892
Blauvelt, D. G., Sato, T. F., Wienisch, M., & Murthy, V. N. (2013). Distinct spatiotemporal activity in principal neurons of the mouse olfactory bulb in anesthetized and awake states. Frontiers in Neural Circuits, 7. http://doi.org/10.3389/fncir.2013.00046
Blaise, J. H. (2013). Long-term Potentiation of Perforant Pathway-dentate Gyrus Synapse in Freely Behaving Mice. Journal of Visualized Experiments, (81), e50642. http://doi.org/10.3791/50642
Wang, H., Siddharthan, V., Kesler, K. K., Hall, J. O., Motter, N. E., Julander, J. G., & Morrey, J. D. (2013). Fatal neurological respiratory insufficiency is common among viral encephalitides. The Journal of Infectious Diseases, 208(4), 573–83. http://doi.org/10.1093/infdis/jit186
Zhang, Q.-X., Lu, R.-W., Curcio, C. A., Yao, X.-C., DC., H., SE., N., … PN, D. (2012). In Vivo Confocal Intrinsic Optical Signal Identification of Localized Retinal Dysfunction. Investigative Opthalmology & Visual Science, 53(13), 8139. http://doi.org/10.1167/iovs.12-10732
Badsha, F., Kain, P., Prabhakar, S., Sundaram, S., Padinjat, R., Rodrigues, V., & Hasan, G. (2012). Mutants in Drosophila TRPC channels reduce olfactory sensitivity to carbon dioxide. PloS One, 7(11), e49848. http://doi.org/10.1371/journal.pone.0049848
Konow, N., Azizi, E., & Roberts, T. J. (2012). Muscle power attenuation by tendon during energy dissipation. Proceedings. Biological Sciences / The Royal Society, 279(1731), 1108–13. http://doi.org/10.1098/rspb.2011.1435
Chen, S., Mohajerani, M. H., Xie, Y., & Murphy, T. H. (2012). Optogenetic analysis of neuronal excitability during global ischemia reveals selective deficits in sensory processing following reperfusion in mouse cortex. The Journal of Neuroscience?: The Official Journal of the Society for Neuroscience, 32(39), 13510–9. http://doi.org/10.1523/JNEUROSCI.1439-12.2012
Morrey, J. D., Siddharthan, V., Wang, H., Hall, J. O., Motter, N. E., Skinner, R. D., & Skirpstunas, R. T. (2010). Neurological suppression of diaphragm electromyographs in hamsters infected with West Nile virus. Journal of Neurovirology, 16(4), 318–329. http://doi.org/10.3109/13550284.2010.501847
Brundage, C. M., & Taylor, B. E. (2010). Neuroplasticity of the central hypercapnic ventilatory response: teratogen-induced impairment and subsequent recovery during development. Developmental Neurobiology, 70(10), 726–35. http://doi.org/10.1002/dneu.20806
Kim, E., Grover, L. M., Bertolotti, D., & Green, T. L. (2010). Growth hormone rescues hippocampal synaptic function after sleep deprivation. American Journal of Physiology. Regulatory, Integrative and Comparative Physiology, 298(6), R1588-96. http://doi.org/10.1152/ajpregu.00580.2009
Kavlie, R. G., Kernan, M. J., & Eberl, D. F. (2010). Hearing in Drosophila requires TilB, a conserved protein associated with ciliary motility. Genetics, 185(1), 177–88. http://doi.org/10.1534/genetics.110.114009
Lenti, L., Domoki, F., Gáspár, T., Snipes, J. A., Bari, F., & Busija, D. W. (2009). N-Methyl- d -Aspartate Induces Cortical Hyperemia through Cortical Spreading Depression-Dependent and -Independent Mechanisms in Rats. Microcirculation, 16(7), 629–639. http://doi.org/10.1080/10739680903131510
Su, C.-K., Ho, C.-M., Kuo, H.-H., Wen, Y.-C., & Chai, C.-Y. (2009). Sympathetic-correlated c-Fos expression in the neonatal rat spinal cord in vitro. Journal of Biomedical Science, 16(1), 44. http://doi.org/10.1186/1423-0127-16-44
Brundage, C. M., & Taylor, B. E. (2009). Timing and duration of developmental nicotine exposure contribute to attenuation of the tadpole hypercapnic neuroventilatory response. Developmental Neurobiology, 69(7), 451–61. http://doi.org/10.1002/dneu.20720
Orem, N. R., Xia, L., & Dolph, P. J. (2006). An essential role for endocytosis of rhodopsin through interaction of visual arrestin with the AP-2 adaptor. Journal of Cell Science, 119(Pt 15), 3141–8. http://doi.org/10.1242/jcs.03052
Markham, M. R., & Stoddard, P. K. (2005). Adrenocorticotropic hormone enhances the masculinity of an electric communication signal by modulating the waveform and timing of action potentials within individual cells. The Journal of Neuroscience?: The Official Journal of the Society for Neuroscience, 25(38), 8746–54. http://doi.org/10.1523/JNEUROSCI.2809-05.2005
Cartford, M. C. (2004). Cerebellar norepinephrine modulates learning of delay classical eyeblink conditioning: Evidence for post-synaptic signaling via PKA. Learning & Memory, 11(6), 732–737. http://doi.org/10.1101/lm.83104
Jonker, D. M., Vermeij, D. A. C., Edelbroek, P. M., Voskuyl, R. A., Piotrovsky, V. K., & Danhof, M. (2003). Pharmacodynamic Analysis of the Interaction between Tiagabine and Midazolam with an Allosteric Model That Incorporates Signal Transduction. Epilepsia, 44(3), 329–338. http://doi.org/10.1046/j.1528-1157.2003.37802.x
van den Pol, A. N., Ghosh, P. K., Liu, R., Li, Y., Aghajanian, G. K., & Gao, X.-B. (2002). Hypocretin (orexin) enhances neuron activity and cell synchrony in developing mouse GFP-expressing locus coeruleus. The Journal of Physiology, 541(1), 169–185. http://doi.org/10.1113/jphysiol.2002.017426
Ransom, C. B., Ransom, B. R., & Sontheimer, H. (2000). Activity-dependent extracellular K+ accumulation in rat optic nerve: the role of glial and axonal Na+ pumps. The Journal of Physiology, 522 Pt 3, 427–42. Retrieved from http://www.pubmedcentral.nih.gov/articlerender.fcgi?artid=2269766&tool=pmcentrez&rendertype=abstract
Alloway, P. G., & Dolph, P. J. (1999). A role for the light-dependent phosphorylation of visual arrestin. Proceedings of the National Academy of Sciences, 96(11), 6072–6077. http://doi.org/10.1073/pnas.96.11.6072
Lipchik, G. L., Holroyd, K. A., France, C. R., Kvaal, S. A., Segal, D., Cordingley, G. E., … McCool, H. R. (1996). Central and peripheral mechanisms in chronic tension-type headache. Pain, 64(3), 467–75. Retrieved from http://www.pubmedcentral.nih.gov/articlerender.fcgi?artid=2128054&tool=pmcentrez&rendertype=abstract
Knuckey, N. W., Palm, D., Primiano, M., Epstein, M. H., & Johanson, C. E. (1995). N-Acetylcysteine Enhances Hippocampal Neuronal Survival After Transient Forebrain Ischemia in Rats. Stroke, 26(2), 305–311. http://doi.org/10.1161/01.STR.26.2.305
Request
Catalogue
Chat
Print