

Microscope Incubators
Developed by Microscopists, for Microscopists: In Vivo Scientific offers the most advanced live cell imaging environments available.
- Overview
- Specifications
- Accessories
- Citations
- Related Products
Overview
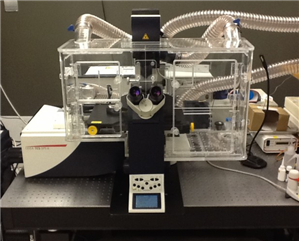
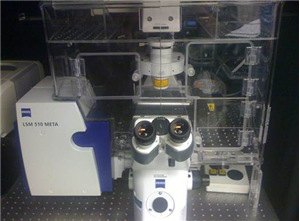
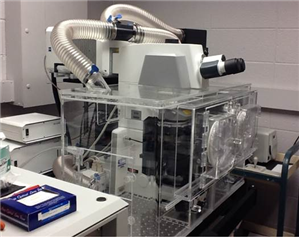
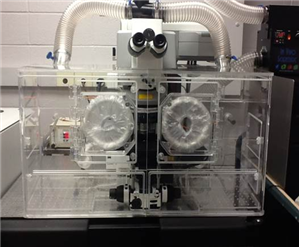
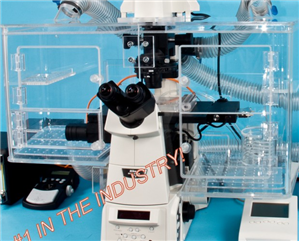
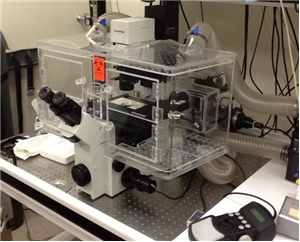
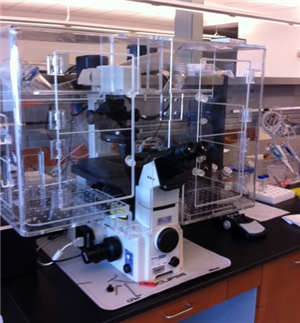
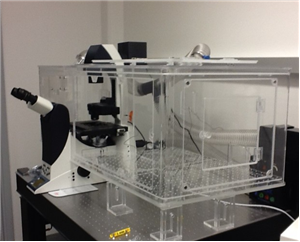
There are 8 images available to view - click to enlarge and scroll through the product gallery.
Live Cell Microscopy
/ Download as PDF
Check also our CELL IMAGING section
In Vivo Scientific offers the most advanced live cell imaging environments available. As all of their products have been designed and empirically tested by scientists working in the live cell microscopy field, they can ensure the absolute highest degree of temperature precision and stability.
Other incubators for live cell microscopy rely on passive, random diffusion of heated air from a single source to maintain the desired temperature setpoint at the sample (left hand image below). Since these systems provide no air-return, this heated air eventually escapes from the incubator through cracks at the microscope/incubator junction in an uncontrolled, random fashion. Because of this design, such incubators suffer from the following flaws:
- Lack of temperature uniformity throughout the chamber and across the stage, causing temperature differences across multiwell plates.
- Temperature flucturations at the sample being imaged, resulting in focus drift.
- Electrical and vibrational interference from the heater.
- Large changes in temperature when the imaging environment is disturbed (e.g. incubator doors opened).
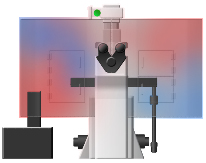
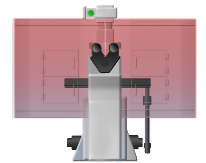
Temperature uniformity of other incubators (left) vs. In Vivo Scientific incubators (right). Warmer temps indicated by red and cooler temps by blue.
In Vivo Scientific incubators are designed and empirically tested to reduce or eliminate these flaws found in other incubators. Instead of relying on passive diffusion of air to warm the incubator/sample, our incubators employ a unique diffusion grid design in combination with both an air input and an air return. This design results in predictable, uniform airflow throughout the chamber, eliminating cool or hot spots within the incubator itself, and vastly improving temperature stability at the sample being imaged. Maintenence of the desired temperature setpoint is achieved through the use of a precision, shielded temperature probe coupled to a peripheral heating unit. Because the heater unit can be placed away from the incubator itself, elimination of electrical and vibrational interference from the heater itself can be achieved. Accuracy +/- 0.1 deg. C can routinely be maintained at the sample itself, and 0.2 deg. C. across the microscope stage (allowing for uniform heating of multiwell dishes). These tight tolerances result in minimal focal drift once sample/incubator equilibrium has been achieved. Furthermore, because of the airflow pattern and temperature uniformity, the incubator temperature rapidly re-adjusts following the opening of incubator doors (e.g. during sample changing/manipulation or microscope hardware manipulation).
Thermal Stability of In Vivo Scientific Incubators. Temperatures were recorded with a digital temperature probe placed at the center of the stage. High precision temperature stability is maintained over both short (left) and long (right) durations.
![]() |
Left: In Vivo Scientific Incubator on a Nikon Eclipse TE-2000 Microsocpe. Incubators for Zeiss and Olympus microscopes are also available, as well as versions that accommodate confocal modules. All incubators are compatible with all commercially available cameras, light sources, filter wheels, motorized stages, and motorized nosepieces.All incubators are designed with ergonomics as well as performance in mind. Microscope controls are easily accessible when the incubator is installed, and focus and x,y stage controls of the microscope are outside of the incubator itself. Large, logically positioned doors allow easy access to the specimen and other microscope controls , and smaller doors allow for cords, tubing, and miscellaneous access. The incubator itself can be installed by a single person in a matter of minutes. |
Frequently Asked Questions
Q: Why do I need a microscope incubator? Won't a heated stage be sufficient?
A: Heated stages are sufficient only for low magnification/N.A., dry objective imaging. This is because small fluctuations in ambient air temperature cause changes in the plane of focus at high magnifications, known as focus drift, causing the sample to move into and out of focus. Furthermore, a lens heater is required if high N.A. immersion objectives are used, since the high thermal conductivity of water/oil coupled with a room-temperature objective causes a drop in temperature of the sample itself (sometimes to almost room temperature itself). Thus, the very cell being imaged is not at physiological temperature, even if the rest of the dish is! However, lens heaters used in combination with heated stages often exhibit precision/stability problems, resulting in uncontrollable focal drift at high magnifications and gradients of temperature across the sample. The advantage of a full microscope enclosure is that all of the imaging components are at the same temperature (sample, objective, and stage). This makes live-cell imaging much easier and more reliable.
Q: How is the microscope incubator made by In Vivo Scientific different from incubators made by other manufactures?
A: Other incubators for live cell microscopy rely on passive, random diffusion of heated air from a single source to maintain the desired temperature setpoint at the sample. In Vivo Scientific incubators are designed and empirically tested to reduce or eliminate these flaws found in other incubators. Instead of relying on passive diffusion of air to warm the incubator/sample, our incubators employ a unique diffusion grid design in combination with both an air input and an air return. This design results in predictable, uniform airflow throughout the chamber, eliminating cool or hot spots within the incubator itself, and vastly improving temperature stability at the sample being imaged. See incubators for more information.
Q: I need the absolute highest level of temperature precision and stability at high magnifications; how should I achieve this?
A: Use an objective insulator in combination with the microscope incubator. Even though the objective is being heated by the surrounding air of the incubator, the microscope body represents a very large heat mass which is difficult to maintain at physiological temperatures (this is an inherent problem with all microscope incubators). As a result, the objective may be a few degrees lower in temperature than the sample itself. For most applications, this does not represent a problem; however, the use of our objective insluator eliminates this problem.
Specifications
Accessories
Citations
Arce, S., Wu, P., & Tseng, Y. (2013). Fast and accurate automated cell boundary determination for fluorescence microscopy. Scientific reports. Retrieved from https://www.nature.com/srep/2013/130724/srep02266/full/srep02266.html
Breckenridge, M., Desai, R., & Yang, M. (2013). Substrates with Engineered Step Changes in Rigidity Induce Traction Force Polarity and Durotaxis. Cellular and Molecular …. Retrieved from https://link.springer.com/article/10.1007/s12195-013-0307-6
Choudhary, A., & Lera, R. (2013). Interphase cytofission maintains genomic integrity of human cells after failed cytokinesis. Proceedings of the …. Retrieved from https://www.pnas.org/content/110/32/13026.short
DiFranco, K., Gupta, A., & Galusha, L. (2012). Leukotoxin (Leukothera®) targets active leukocyte function antigen-1 (LFA-1) protein and triggers a lysosomal mediated cell death pathway. Journal of Biological …. Retrieved from https://www.jbc.org/content/287/21/17618.abstract
Fuller, C., & Straight, A. (2012). Imaging nanometre-scale structure in cells using in situ aberration correction. Journal of Microscopy. Retrieved from https://onlinelibrary.wiley.com/doi/10.1111/j.1365-2818.2012.03654.x/full
Gu, Y., Chen, X., Lee, J., Monteiro, D., Wang, H., & Lee, W. (2012). Inkjet printed antibiotic-and calcium-eluting bioresorbable nanocomposite micropatterns for orthopedic implants. Acta Biomaterialia. Retrieved from https://www.sciencedirect.com/science/article/pii/S1742706111003503
Hennigan, R., Moon, C., & Parysek, L. (2012). The NF2 tumor suppressor regulates microtubule-based vesicle trafficking via a novel Rac, MLK and p38SAPK pathway. Oncogene. Retrieved from https://www.nature.com/onc/journal/vaop/ncurrent/full/onc2012135a.html
Jin, H., Yu, Y., Chrisler, W., & Xiong, Y. (2012). Delivery of microRNA-10b with polylysine nanoparticles for inhibition of breast cancer cell wound healing. Breast cancer: basic and …. Retrieved from https://www.ncbi.nlm.nih.gov/pmc/articles/PMC3256732/
Kandaswamy, U., & Rotman, Z. (2012). Automated condition-invariable neurite segmentation and synapse classification using textural analysis-based machine-learning algorithms. Journal of neuroscience …. Retrieved from https://www.sciencedirect.com/science/article/pii/S0165027012004785
Kim, A., Zhou, C., Lakshman, N., & Petroll, W. (2012). Corneal stromal cells use both high-and low-contractility migration mechanisms in 3-D collagen matrices. Experimental cell research. Retrieved from https://www.sciencedirect.com/science/article/pii/S001448271100499X
Kim, B., Hannanta-Anan, P., & Chau, M. (2013). Cooperative Roles of SDF-1α and EGF Gradients on Tumor Cell Migration Revealed by a Robust 3D Microfluidic Model. PloS one. Retrieved from https://dx.plos.org/10.1371/journal.pone.0068422
Kyburz, K., & Anseth, K. (2013). Three-dimensional hMSC Motility within Peptide-Functionalized PEG-Based Hydrogel of Varying Adhesivity and Crosslinking Density. Acta biomaterialia. Retrieved from https://www.sciencedirect.com/science/article/pii/S174270611300041X
Lee, J., Gu, Y., Wang, H., & Lee, W. (2012). Microfluidic 3D bone tissue model for high-throughput evaluation of wound-healing and infection-preventing biomaterials. Biomaterials. Retrieved from https://www.sciencedirect.com/science/article/pii/S0142961211012403
Lera, R., & Burkard, M. (2012). High mitotic activity of Polo-like Kinase 1 is required for chromosome segregation and genomic integrity in human epithelial cells. Journal of Biological Chemistry. Retrieved from https://www.jbc.org/content/287/51/42812.short
Marley, A., Choy, R., & Zastrow, M. von. (2013). GPR88 Reveals a Discrete Function of Primary Cilia as Selective Insulators of GPCR Cross-Talk. PloS one. Retrieved from https://dx.plos.org/10.1371/journal.pone.0070857
McGrail, D., Ghosh, D., Quach, N., & Dawson, M. (2012). Differential mechanical response of mesenchymal stem cells and fibroblasts to tumor-secreted soluble factors. PloS one. Retrieved from https://dx.plos.org/10.1371/journal.pone.0033248
McGrail, D., McAndrews, K., & Dawson, M. (2012). Biomechanical analysis predicts decreased human mesenchymal stem cell function before molecular differences. Experimental cell research. Retrieved from https://www.sciencedirect.com/science/article/pii/S0014482712004740
Misko, A., Sasaki, Y., & Tuck, E. (2012). Mitofusin2 mutations disrupt axonal mitochondrial positioning and promote axon degeneration. The Journal of …. Retrieved from https://www.jneurosci.org/content/32/12/4145.short
Peng, A., Rotman, Z., Deng, P., & Klyachko, V. (2012). Differential motion dynamics of synaptic vesicles undergoing spontaneous and activity-evoked endocytosis. Neuron. Retrieved from https://www.sciencedirect.com/science/article/pii/S0896627312001419
Qiao, D., Meyer, K., & Friedl, A. (2013). Glypican-1 Stimulates S Phase Entry and DNA Replication in Human Glioma Cells and Normal Astrocytes. Molecular and cellular biology. Retrieved from https://mcb.asm.org/content/early/2013/09/05/MCB.00238-13.abstract
Sakurai, T., & Tsuchida, M. (2013). Cardiomyocyte FGF signaling is required for Cx43 phosphorylation and cardiac gap junction maintenance. Experimental cell research. Retrieved from https://www.sciencedirect.com/science/article/pii/S0014482713002280
Sen, S., Ng, W., & Kumar, S. (2012). Contributions of talin-1 to glioma cell–matrix tensional homeostasis. Journal of The …. Retrieved from https://rsif.royalsocietypublishing.org/content/9/71/1311.short
Stehbens, S., & Pemble, H. (2012). Imaging intracellular protein dynamics by spinning disk confocal microscopy. Methods in …. Retrieved from https://www.ncbi.nlm.nih.gov/pmc/articles/PMC3495241/
Wadhawan, N., Kalkat, H., & Natarajan, K. (2012). Growth and positioning of adipose-derived stem cells in microfluidic devices. Lab Chip. Retrieved from https://pubs.rsc.org/en/content/articlehtml/2012/lc/c2lc40891k
Wang, Y., Zhang, C., & Wei, N. (2012). Experimental Study of Crossover from Capillary to Viscous Fingering for Supercritical CO2–Water Displacement in a Homogeneous Pore Network. … science & technology. Retrieved from https://pubs.acs.org/doi/abs/10.1021/es3014503
Yadav, S., Puthenveedu, M., & Linstedt, A. (2012). Golgin160 recruits the dynein motor to position the Golgi apparatus. Developmental cell. Retrieved from https://www.sciencedirect.com/science/article/pii/S153458071200250X
Yeh, T., Kowalska, A., & Scipioni, B. (2013). Dynactin helps target Polo-like kinase 1 to kinetochores via its left-handed beta-helical p27 subunit. The EMBO …. Retrieved from https://www.nature.com/emboj/journal/v32/n7/abs/emboj201330a.html
Zhang, W., Soman, P., & Meggs, K. (2013). Tuning the Poisson’s Ratio of Biomaterials for Investigating Cellular Response. Advanced Functional …. Retrieved from https://onlinelibrary.wiley.com/doi/10.1002/adfm.201202666/full
Request
Catalogue
Chat
Print